Peak Oil, Climate Change and Local Sustainability
Peak oil is the theory that we have or are about to have used up half the world’s endowment of oil and that what oil remains will be increasingly scarce and unavailable, steadily poorer quality, and increasingly more expensive. IT IS NOT a theory that we are about to run out of oil.
Global warming is the theory that man-made atmospheric pollution, mostly from the burning of fossil fuels, is causing the earth’s atmosphere to heat up to potentially dangerous levels.
Global Dimming is the theory that air born particulates, mostly from man-made pollution, are reducing the amount of the sun’s light and energy that are reaching the earth’s surface.
Overpopulation is the theory that total human population is exceeding the real carrying capacity of the planet.
I could speak for a whole hour on any one of those looming crises, but I won’t. My purpose here this evening is to try to encapsulate in a half hour or so how in this century the merger and interrelationship of those four crises, and a few other peripheral and related crises such as water, are going to affect;
* Food Security,
* Carrying capacity,
* Soil Fertility,
* Sustainability,
Both globally and, more important, locally here in Ontario and in Prince Edward and surrounding counties.
A few words about energy consumption;
* Global energy use per capita has risen more than 10 fold since the start of 20th century.
* One energy source has not replaced another, each is treated as an add on to the energy mix, resulting in layered energy options.
* Each of us uses the energy equivalent of over 300 slaves.
* Food on your table has traveled an average of over 2500 kilometers.
* The greater the energy use, the further removed we get from sustainability and self-sufficiency.
* This century we will hit not only peak oil but peak natural gas, peak coal, peak uranium, peak plutonium, as well as peaks in dozens of other finite resources; copper, gold, nickel, selenium, zinc, molybdenum, & more.
See my blog articles,
Energy as the Catalyst in the Punctuated Equilibrium of Human Population Growth and
Alternative Energy, Add-ons and ReplacementsThere is a not so old Saudi saying that prophetically encapsulates what we are facing in the near future with energy. It goes;
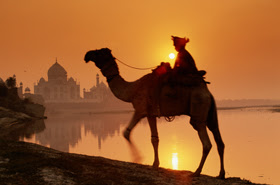
"My father rode a camel. I drive a car.
My son flies a jet-plane.
His son will ride a camel."
The most critical and dangerous dependence on fossil fuels we have built is in food production and distribution. We use an average of 10 calories of energy, mostly from fossil fuels, to produce 1 calorie of food.
Despite the fact that we share it, albeit reluctantly, with millions of other species, the human population monopolizes over ¼ of the primary food production capacity of the planet. There is obviously considerable capacity still available to us, but only if we want to be alone. Our increasing monopoly of that primary productive capacity directly parallels the increase in human-caused species extinction. That doesn’t make us particularly evil. The dominant species in any environment always takes what it needs leaving weaker competition to fight for the scraps.
For the moment, then, let’s forget about those millions of other species. What exactly is the carrying capacity of the planet?
* Of the total global land mass, about 35% is arable land, 31% forested and 34% is either desert, tundra/permafrost or dedicated to other human uses such as urbanization or activity such as mining or recreation.
* Globally there are about 7.8 billion acres that are potentially arable of which 3.5 billion acres are now being used to produce food.
* The unused potential land is generally in areas lacking essential transport for moving product to market or infrastructure needed for the support of commercial food production.
* Of the 35% of the total global land mass that is arable or suitable for agriculture, about 2/3 is pasture or meadowland used for livestock.
* Another 10% (about 350 million acres) is used for grain and cereal production, much of that (75% or about 270 million acres) also used for livestock.
* Despite the global attraction of and growth in permaculture, only 1 percent of arable land is dedicated to permanent crops such as fruits and nuts.
* Essentially, all of human food production, excluding meat, dairy and sea food, is grown on less than 400 million acres. Each of those acres is, therefore, feeding about 15-17 people.
* If we were to bring all of the unused arable land into agricultural production with the same patterns as at present (85%+ for livestock support) we would net very little additional food-production land (about 600 million acres) to offset the anticipated drop in productivity of 80-90% on those current 400 million acres following peak oil. This would barely allow us to absorb the impact of a 25% drop in productivity.
* The unused potentially arable land is not in the same place as the 6.6 billion of us for whom it could reduce the impact of soil productivity loss following peak oil. It exists in pockets within the boundaries of already sovereign nations throughout the world, nations which themselves may be impoverished and unable to currently produce enough food for their own people.
* Probably, based on current patterns, these lands would more likely be used for high-profit crops like corn, soy and sugar cane to be used to produce bio-fuels in a world increasingly deficient in fossil fuel energy.
See my blog article,
Post-Peak Agricultural CapacityGlobal Water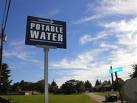
We are very fortunate in Canada, exceptionally so in southern Ontario. Canada in general and southern Ontario in particular should be able to avoid the fresh water issues that will face much of the rest of the world this century and in the foreseeable future. The water rights issues that plague many drier areas such as Africa, South Asia and the western U.S. are and will be only an occasional problem in this area.
* Canada has the third highest volume of renewable fresh water in the world behind Brazil and Russia.
* Canada has more lake area than any other country in the world.
* The Great Lakes are the largest system of fresh, surface water on earth, containing roughly 18 percent of the world supply.
* Ninety-nine percent of surface freshwater by volume is in lakes and only one percent in rivers.
That is not to suggest that anyone should be complacent about water. Ultimately fresh water availability nets down to a resource issue for a single property, a single family, a single community. In essence, if you need it and you don’t have it or don’t have access to it, it is a serious problem for you.
* More than 2,200 major and minor water-related natural disasters occurred in the world between 1990 and 2001. Asia and Africa were the most affected continents, with floods accounting for half of these disasters.
* Of the dozens of water rights conflicts around the world I have read about and researched almost always agricultural water needs and water rights have lost out to the demands of urban areas and industry.
* Whenever the resolution of water rights conflicts is left in the hands of politicians and bureaucrats the resolution will be decided in favour of the highest voting density and financial contribution.
* The U.S. are moving inexorably toward solving the water needs of their agricultural and industrial heartland through the southward diversion of the waters of the Great Lakes. Considering the massive engineering project this would involve, peak oil could be a blessing in disguise.
Statistics from
How much do we have?Continued net decline in soil productive capacity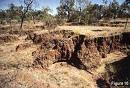
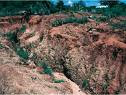
We are systematically destroying the overall fertility of our soil and losing productive soil.
* Our use of agricultural pesticides kills critical soil organisms,
* Intensive deforestation results in losing billions of tons of top soil,
* Annual U.S. topsoil loss is 7 billion tons (23 tons/year/person, 85% directly attributable to raising livestock),
* Intensive irrigation leaches vital mineral content out of topsoil,
* Air pollution results in toxic chemicals being absorbed into the soil,
* Industrial scale plowing and tilling results in billions of tons of top soil being dried up and blown away every year,
* We create an impermeable layer of hardpan just below the top layer of soil which prevents both plant roots and soil microorganisms reaching the mineral nutrients in the subsoil,
* Turning the soil exposes deep topsoil organisms and drives aerobic microorganisms deeper underground where they are killed for lack of air, water and heat from sunlight,
* We systematically destroy balance of soil nutrients through continued application of artificial NPK fertilizers,
* We change soil ph levels making it inhospitable for many soil organisms,
* We change symbiotic relationship of soil organisms and plants by replacing native plants with chemical-dependant monoculture.
* Soil erosion and other forms of land degradation now rob the world of 70-140,000 km2/year of farming land.
* Urbanization alone is responsible for the loss of 20-40,000km2/year.
* Worldwide, soil erosion has caused abandonment of 4.3 million km2 of arable land during the last four decades.
* The total world availability of topsoil is estimated at 7,000 gigatonnes - about seventy years of topsoil at current rates of destruction and loss.
State of global food production, consumption, distribution* Before the Industrial Revolution the majority of people produced most of their own food, most of the rest acquired within a few miles of home.
* People then ate what could grow locally and ate what was in season or that they stored/preserved themselves. Now we eat tomatoes and celery in February, kiwi fruit and spring lamb from New Zealand, oranges from Israel, rice from the far east, beef from Argentina.
* The vast majority of people in the developed (and increasingly in the developing) world today who are growing food (now less than 2% of population) are not doing so for their own consumption.
* Agricultural produce today is fed into a heavily fossil-fuel dependant global food distribution system.
* Food today travels an average of 2500+ kilometers before reaching your table, takes a week, often much longer, to get there.
* Average food miles continue to grow with spread of urbanization and corporate-driven regional crop concentration.
* Food grown locally will be available in local food stores only by chance after having worked through the distribution system.
* Today more than ½ of global population live in cities, most with severe restrictions standing in the way of producing their own food.
* Most cities originated in and continue to expand into the very farmland they need to produce the food needed by their populations (urbanization responsible for loss of 20-40,000km2/year of arable land).
* The majority of people engaged in agriculture today are working land they do not own to produce product they do not, and cannot afford to, consume themselves.
* About 85% of “productive” agricultural land today is devoted to the support of livestock.
* The average diet today contains more meat and animal products in one day than our ancestors consumed in a week.
* Global emergency food grain reserves have shrunk in past ten years from marginal 119 day supply to a sub-critical 53 day supply.
* Ever increasing proportion of global food coming from GMO crops, an increasing use of terminator genes to ensure ongoing seed co. sales.
* Multinational corporations decide what is grown, how its grown, what is available in your local market (stocking decisions not made locally).
Understanding plant nutrition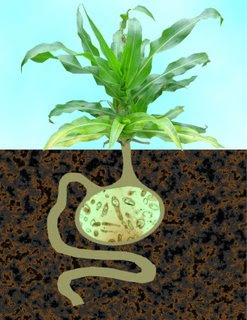
Plants receive their nutrients through and digest their food, like you, in their stomachs. The topsoil in which a plant grows is, in reality, its stomach. The stomach is an organism’s nutrient supply buffer between the outside world and the critical internal systems. Whole foods are broken down by bacteria and enzymes into pure form suitable for transport through those internal systems, like our blood stream, to the cells and organs which need those nutrients for metabolism and maintenance. Enzymes intercept toxins and eliminate them before they can enter those internal systems. A glut of nutrients is buffered and released slowly to those internal systems.
For a plant, all of these functions happen external to its critical internal systems. Bacteria and enzymes in the topsoil, especially that narrow band of topsoil immediately around the roots, the root zone, do this work. The roots themselves, to continue the parallel, are like the portal vein leading from the small intestine to the liver. It is the last part of the digestive system external to the organism’s sensitive internal systems. The root zone is like the small intestine, releasing the broken down essential nutrients to the roots (the portal vein) for absorption into the internal systems.
Just as the digestion in your stomach is under the control of your internal systems (principal digestive enzymes are generated in your pancreas and released into the stomach) the “digestion” for a plant in the soil is under the control of the plant in that the principal digestive enzymes are generated internally and released into the root zone.
See my blog articles,
Plants with stomachs - Peak oil implications and
Plant stomachs and animal stomachs: the differences and similaritiesTopsoil as a living organism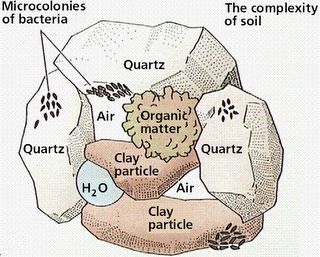
Just like your skin is the largest organ of your body, the topsoil may be the largest living organism on the planet.
A single pound of soil contains tens of millions of bacteria, fungi and other soil microorganisms critical to the survival of all terrestrial life. Many of these microorganisms can trace their family tree (pardon the pun) all the way back to the very beginning of life on earth.
Nature wastes nothing. Those microorganisms are responsible for breaking down the raw resources in the soil and beginning the process of cycling it through the web of life, whether that resource be a fallen tree, a dead animal, a pile of elephant dung, a cow’s placental sack, or the minerals in a rock that has worked its way up from the subsoil.
We have, unfortunately, put a large portion of our planet’s topsoil on force-fed chemical life-support with our use of artificial fertilizers, pesticides, herbicides and other agrichemicals, like a brain-dead patient on intravenous life-support. We are destroying the natural fertility of the soil in the process. Just as it takes time to put a coma-recovery patient back on solid food (like weaning an infant off the bottle), it will take considerable time, possibly decades, to restore the natural fertility of soil that has been chemically maintained for decades.
When we remove life-support from the coma patient that patient generally dies relatively quickly. What will happen to our agricultural productivity when we pass peak oil and the agrichemicals begin to disappear? When we pull the plug on our soil’s artificial life-support?
Building/Maintaining Natural Soil Fertility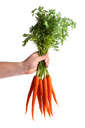
You’ve just picked a bunch of carrots. Consider what they are composed of;
* Water from the soil (maybe from rain or maybe irrigation),
* Hydrogen (in the carbohydrates) mostly from the water,
* Carbon, partly from CO2 but also from the soil,
* Other minerals like iron, sulfur, phosphorus, potassium from the soil,
* Fiber, collagen and vitamins like beta carotene produced by the plant from nutrients derived from the soil.
For most people the top of the plant ends up as “plant waste“. Before the carrot is eaten the root hairs and possibly the skin is pealed, more plant waste. The carrots are washed, the dirt flushed away. The carrot may be cooked, many nutrients lost into the cooking water which is discarded.
Generally none of this “wasted” plant material makes its way back to the soil in which the carrot was grown. The entire mass of that plant that was derived from the soil is a net loss for that soil.
We cannot continue to take organic material from the soil and replace it with a limited range of artificial nutrients (NPK) and expect that soil to remain viable. If we lose 100 billion tons of topsoil every year through erosion, how many billion tons or topsoil mass do we lose every year in food that we harvest from it? Artificial fertilizer use has grown 33-fold over the course of the Green Revolution in an unsuccessful attempt to maintain crop yields. And that usage will continue to grow, and still not maintain topsoil mass.
If we do not begin or resume returning to the soil what we take from it, we will continue to deplete the topsoil at a far greater rate than nature alone can replace it. Just as with any other resource, if we use it faster than it is being replaced it can eventually run out. We have broken the cycle. We must reconnect it.
As you drive around country roads take a look at the fields that have been in long term use for producing cash crops. Compare the depth of the soil in those fields to the depth of that surrounding those fields. The difference is mostly a combination of three things;
1) Soil compaction through the use of heavy farm equipment;
2) Soil loss from wind and water erosion;
3) Most important, continual loss of soil mass from crops being harvested and the “waste” organic matter not being returned to the soil.
I’m not sure if there are any examples around here but around cities like Toronto, when they build a subdivision, the first thing they do is strip all the top soil and pile it up in a big hill. From there it may be sold to a jobber to be “cleaned”, bagged and sold in garden centers.
When the homes are completed the top soil that was stripped (it may have been a foot deep) is replaced with grass sod that may have as much as a whole inch of soil held by the grass roots. Homeowners go to the garden center to buy bags of topsoil (it may have actually come from the land on which their house sits) to build their flower gardens.
On the other side of peak oil when the global food distribution system begins to break down, the chance of growing any significant amount of food on suburban plots is slim and none.
Soil fertility consists of a number of key factors; carbon, other trace minerals, organic content, humus, soil microorganisms, water.
Liebig’s Law of the Minimum says that growth is controlled not by the total resources available but by the scarcest resource. When a plant consists primarily of carbon and carbon-based molecules, how can we believe that fertilizing with Nitrogen, Phosphorus and Potassium is going to allow us to maintain yields and soil mass?
You restore and maintain natural soil fertility by building and continuing to nurture and replenish; carbon, other trace minerals, organic content, humus, and soil microorganisms.
Carbon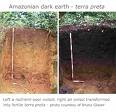
There is a soil type in the Amazon region of South America that has been receiving considerable scientific attention. It is called Terra Preta soil. It has been determined that this soil, as it is now constituted, is man-made. There are two keys to Terra Preta soil; 1) high carbon content, 2) a unique community of soil bacteria. The carbon in Terra Preta soil, it has been determined, originates from charcoal created as part of an ancient slash and char method of soil preparation. Terra Preta soil will net a yield of 300-800% that achievable in adjacent non-Terra Preta soil. Though it is thought that the unique soil bacteria is the more important component, black carbon soil supplementation alone has been proven to be very beneficial to soil and significantly improve yields, shorten required fallow times, improve soil water retention, reduce soil toxicity levels, and encourage growth of beneficial soil microorganism populations. Terra Preta soil, by the way, has also been found and proven to be self-renewing.
See the articles
Terra Preta Soils - Agricultural Miracle from the Past? and
Origins of Amazonia's Terra Preta Soils in my blog
Other trace mineralsThe minerals in the soil largely originate from the base rock below the soil. That base rock is very slowly broken down by plant roots and soil microbes. In a natural system the minerals from the soil are constantly recycled by living organisms. In our unnatural agricultural systems minerals taken up by plants (they are used as critical co-factors in enzymes and hormones, in the formation of chlorophyll, in the structure of vitamins and more) is lost to the soil as those plants are removed in harvesting. Organic farmers often dust their fields with rock dust and other sources of trace minerals to replace minerals removed from the soil in harvesting. Bags of these materials can generally be purchased at garden centres and farm supply outlets.
Organic content and Soil MicroorganismsThere are, of course, several traditional ways to return organic matter to the soil; using manure fertilizers, fallowing, planting and plowing under green manure crops, and composting. With the demands for maximum crop yields most of these practices have been abandoned in commercial farming operations. This is the most critical change that has to occur in revitalizing our soil fertility. Critically important is that all of these practices have a dramatic impact on the growth and health of the population of soil microbes. Organic content in the soil is also the most critical factor in the ability of the soil to maintain moisture, thus minimizing demands for irrigation.
See my blog article
Soil fertility and carrying capacityPost-peak Community versus homestead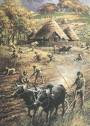
The vast majority of people who become aware of the peak oil issue soon begin to consider the question of what type and size of community they want to be part of beyond peak oil, which communities will be the most sustainable, self-sufficient, self-reliant and survivable in the future.
The answer to that question is tremendously variable for a number of factors such as climate but most particularly depending on how far into the future one is thinking.
I cannot, in the time I have available, do justice to this issue. I would strongly suggest that you see a number of articles on my blog, Oil, Be Seeing You dealing with the issue;
* An argument against personal peak-oil preparation
* Homestead or community?
* Is there any alternative to powerdown? And the sooner the better?
* The right to pursue powerdown: seeking alternative lifestyles post-peak
* Relocalization and retail food chainsEssentially my belief is that it is critical to build strong, viable, self-sufficient and self-reliant community in preparation for the fast-approaching post-peak world. That community and its self-reliance critically includes all agricultural efforts in and around the community. The type of world in which our ancestors scratched self-reliant homesteads from the wilderness simply doesn’t exist anymore. The feeling for it may be strong in certain individuals but it has entered the world of myth.
Ideally post-peak community focuses on a bio-region. As I mentioned earlier, Prince Edward County is a very viable natural bio-region. The prospects of creating the self-reliance and self-sufficiency that will be the hallmark of successful post-peak communities is very strong here.
* There has been a strong build-up of a community of artisans and craft-persons with the type of skills that will be strong components of a post-fossil-fuel community.
* The county is effectively surrounded by water resources that should remain viable into the future.
* The Trent water system continues to deliver vital soil nutrients.
* The climate of the region is tempered by the water surrounding it.
* There is a strong agricultural tradition in the county, as well as a significant core of organic agriculture.
* The sizes of all communities in the county is conducive to building a regional network of self-reliant communities with a rational distribution of specialized skills and crafts.
The type of community that will be suited to the post-peak world, however, does not just happen. In our modern world the feeling and spirit of community, even here, has largely been lost. It will be critical to determine the skill sets that will be needed and ensure that those skills are built.
I could go on at length on this subject but it is important that you address this. The community has to be built from within. Local initiatives like the proposed establishment of a local farmer’s market, which I urge you to support, will be what makes the difference.
What you can/should do to prepare for the future* Take care of whatever soil you have access to, maintaining soil fertility
* Ensuring access to clean water
* Understand sustainability and stewardship
* Forest management, plant trees, preferably hardwood, replace any harvested trees in kind
* Soil testing, classification, integration
* Root cellars, dehydrating, canning, smoking, drying, freezing
* Support local food production, 100 mile diet, 50 mile diet
* Heritage seeds, seed saving, surpluses
* Permaculture, three sisters, companion planting and other food production techniques
* Big and small islands - Bioregionalism and it's local applicability, relocalization
* Evaluate potential applicability of local currency
* Reduce consumption of animal products: meat and dairy especially; indoor food growing; public education and information exchange on sustainability within the community